WORM HOLES
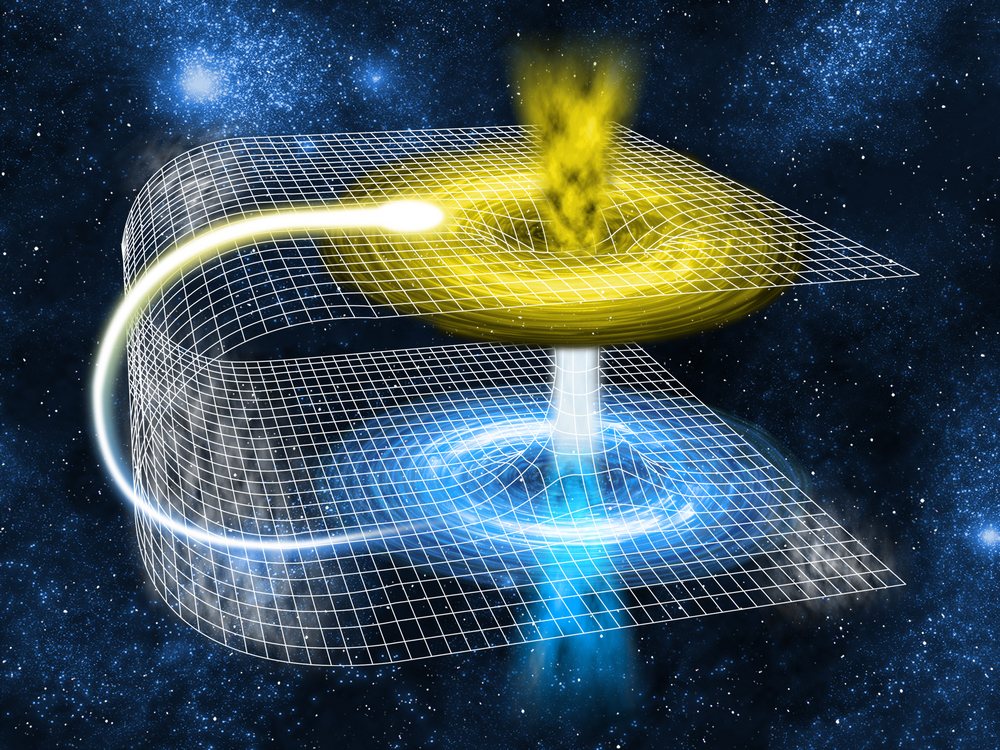
A wormhole is a theoretical passage through space-time that could create shortcuts for long journeys across the universe. Wormholes are predicted by the theory of general relativity. But be wary: wormholes bring with them the dangers of sudden collapse, high radiation and dangerous contact with exotic matter.
In 1935, physicists Albert Einstein and Nathan Rosen used the theory of general relativity to propose the existence of "bridges" through space-time. These paths, called Einstein-Rosen bridges or wormholes, connect two different points in space-time, theoretically creating a shortcut that could reduce travel time and distance.
Einstein's theory of general relativity mathematically predicts the existence of wormholes, but none have been discovered to date. A negative mass wormhole might be spotted by the way its gravity affects light that passes by.
Certain solutions of general relativity allow for the existence of wormholes where the mouth of each is a black hole. However, a naturally occurring black hole, formed by the collapse of a dying star, does not by itself create a wormhole.
Science fiction is filled with tales of traveling through wormholes. But the reality of such travel is more complicated, and not just because we've yet to spot one.
The first problem is size. Primordial wormholes are predicted to exist on microscopic levels, about 10–33 centimeters. However, as the universe expands, it is possible that some may have been stretched to larger sizes.
Another problem comes from stability. The predicted wormholes would be useless for travel because they collapse quickly. But more recent research found that a wormhole containing "exotic" matter could stay open and unchanging for longer periods of time.
Exotic matter, which should not be confused with dark matter or antimatter, contains negative energy density and a large negative pressure. Such matter has only been seen in the behavior of certain vacuum states as part of quantum field theory.
If a wormhole contained sufficient exotic matter, whether naturally occurring or artificially added, it could theoretically be used as a method of sending information or travelers through space.
"A wormhole is not really a means of going back in time, it's a short cut, so that something that was far away is much closer," NASA's Eric Christian wrote.
Although adding exotic matter to a wormhole might stabilize it to the point that human passengers could travel safely through it, there is still the possibility that the addition of "regular" matter would be sufficient to destabilize the portal.
Today's technology is insufficient to enlarge or stabilize wormholes, even if they could be found. However, scientists continue to explore the concept as a method of space travel with the hope that technology will eventually be able to utilize them.